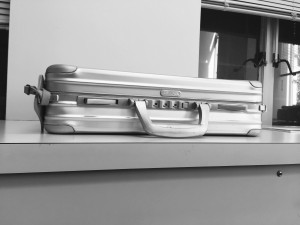
Product liability lawyers should be familiar with both the dangers and the science of steel manufacturing. Steel is one of the most indispensable products in the modern world. Its uses, forms, and composition are limitless. Like any other product, steel in its final form and use is a “product” subject to the same consumer expectation test in Oregon that applies to household appliances. However, unlike most other product manufacturing, steel production, which creates the base material for pipe, rails, aviation, and innumerable transportation, mining, oil and gas, and other products, is incredibly dangerous. Although serious burns might be the most obvious risk, there are also crush, amputation, and a host of other potential injuries which justify the most careful training, exacting safety processes, and best PPE. This is especially true given the danger posed by the typical 24-hour-a-day production schedules and the undisputed fact that nighttime workers are in more danger than day workers.
Steelmaking Is An Ancient Art
In the ancient world, steelmaking was considered an art, and as the centuries passed, the process became more and more complex. Steel was known in antiquity and may have been produced by managing bloomeries, or iron-smelting facilities, in which the bloom contained carbon. Blooms are steel formed into large blocks to which further tempering or chemical procedures can be applied. The use of blooms persists into the steelmaking of today.
The earliest known example of steel production, thought to be about 4000 years old, is a piece of ironware excavated from an archaeological site in Anatolia (the Asian part of Turkey). “Ironware piece unearthed from Turkey found to be oldest steel.” The Hindu (Chennai, India). The Haya people of East Africa invented a type of furnace that they used to make carbon steel at 3,276 degrees Fahrenheit nearly 2000 years ago. Africa’s Ancient Steelmakers (http://www.time.com/time/magazine/article/0,9171,912179,00.html?promoid=googlep). Time Magazine September 25, 1978.
What Is Steel?
Steel is an alloy of iron and carbon. Steelmaking is the process of producing steel from iron and ferrous scrap. In steelmaking today, impurities such as silicon, phosphorus, and excess carbon are removed from the raw iron, and alloying elements such as manganese, nickel, chromium, and vanadium are added to produce different grades of steel. Limiting dissolved gasses such as nitrogen and oxygen, and entrained impurities or “inclusions,” in the steel is also important to ensure the quality of the products cast from the liquid steel. B. Deo and R. Boom, Fundamentals of Steelmaking Metallurgy, Prentice and Hall (1993).
Carbon is the primary alloying element, and its content in steel is between 0.002% and 2.1% by weight. Additional elements are also present in steel, including manganese, phosphorous, sulfur, silicon, and traces of oxygen, nitrogen, and aluminum. Carbon and other elements act as hardening agents, preventing dislocations in the iron atom crystal lattice from sliding past one another.
Varying the amount of alloying elements and the form of their presence in the steel (solute elements precipitated phase) controls qualities such as the hardness, ductility, and tensile strength of the resulting steel. Steel with increased carbon content can be made harder and stronger than iron, but such steel is also less ductile than iron. Ashby, Michael F. and Jones, David R.H. Engineering Materials 2 (with corrections ed.) Oxford: Pergamom Press. ISBN 0-08-032532-7 (1992 [1986]).
Alloys with a higher than 2.1% carbon content are categorized as cast iron. Because cast iron is not malleable even when hot, it can be worked only by casting, where it has a lower melting point. Steel is also distinguishable from wrought iron, which may contain a small amount of carbon.
Even in the narrow range of concentrations that make up steel, mixtures of carbon and iron can form a number of different structures with very different properties. One of the most important polymorphic forms of steel is martensite, a metastable phase that is significantly stronger than other steel phases. When the steel is in an austenitic phase and then quenched rapidly, it forms into martensite, as the atoms “freeze” in place when the cell structure changes from FCC to BCC. Depending on the carbon content, the martensitic phase takes different forms. Below approximately 2% carbon, it takes a ferrite BCC crystal form, but at a higher carbon content, it takes a body-centered tetragonal (BCT) structure. There is no thermal activation energy for the transformation from austenite to martensite. Moreover, there is no compositional change to the atoms, which generally retain their same neighbors. Smith, William F., Hashemi, Jared, Foundations of Materials Science and Engineering (4th ed 2006) McGraw Hill ISBN 0-07-295358-6.
Special Modern High Performance Alloys
There are a number of extremely complex super-alloys and other metals available today for high performance aviation and other uses, including Transformation Induced Plasticity (TRIP) steel and Twinning Induced Plasticity (TWIP) steel. A complete discussion of these super-alloys merits a separate article, and one will be forthcoming shortly.
Introduction To The Modern Process
In the modern era, there are two major processes for making steel. The first is basic oxygen steelmaking, which uses liquid pig iron from the blast furnace and scrap steel for the main feed materials. Alternatively, iron ore is reduced or smelted with coke and limestone in the blast furnace, producing molten iron that is either cast into pic iron or carried to the next stage as molten iron. In the second stage, impurities such as sulfur, phosphorus, and excess carbon are removed, and the alloying elements such as manganese, nickel, chromium, and vanadium are added to produce the steel required. The vast majority of steel in the world is produced using the basic oxygen furnace. In 2011, approximately 70% of the world’s steel was produced in this way. R. Fruehan, The Making, Shaping and Treating of Steel (11th ed. AIST 1999).
The second major modern process is electric arc furnace (EAF) steelmaking, which either uses scrap steel or direct reduced iron (DRI) as the main feed material. Oxygen steelmaking is fuelled predominantly by the exothermic nature of the reactions inside the vessel, whereas in EAF steelmaking, electrical energy is used to melt the solid scrap and/or DRI materials.
In recent times, EAF steelmaking technology has moved closer to Oxygen steelmaking as more chemical energy is introduced into the process. E.T. Turkdogan, Fundamentals of Steelmaking, IOM (1996). EAF steelmaking is predominantly used for producing steel from scrap and involves melting scrap, and combining it with iron ore.
Alternatively, the oxygen method can involve melting DRI using electric arcs (either AC or DC). It is common to start the melt with a “hot heel” (molten steel from a previous heat) and use gas burners to assist with the meltdown of the pile of scrap. EAF furnaces typically have capacities of around 100 tons every 40 to 50 minutes.
Regardless of the process used, through casting, hot rolling and cold rolling, the steel mill then turns the molten steel into blooms, ingots, slabs, and sheet.
At the typical steel mill, the raw materials are batched into a blast furnace where the iron compounds in the ore give up excess oxygen and become liquid iron. At intervals of a few hours, the accumulated liquid iron is tapped from the blast furnace and either cast into pig iron or directed to other vessels for further steelmaking operations. During the casting process, various methods are used, such as the addition of aluminum so that impurities in the steel float to the surface where they can be cut off the finished bloom.
Conclusion
The steelmaking process involves exposure to hundreds of tons of molten metals, often poured manually into ceramic, wax, or other casting forms or hot rolled into shapes. The potential for catastrophic injury or death is everywhere in the steelmaking process, and it is essential that workers be trained and supervised to avoid lapses in safety that could result in such unfortunate occurrences. Although automation continuously decreases the exposure of workers to significant injury or death as a result of virtually every phase of the process, the utmost care should still be exercised by all who enter a steel mill.